Pyruvate dehydrogenase (PDH)
Pyruvate dehydrogenase is a complex enzyme involved in the conversion of pyruvate, a product of glycolysis, into acetyl-CoA, a crucial molecule in energy production and intermediary metabolism.
Table of Contents
Jump to a section:
What is Pyruvate Dehydrogenase?
Pyruvate dehydrogenase (PDH) is a vital 9.5 MDa enzyme complex involved in cellular metabolism. It converts pyruvate, a product of glycolysis, into acetyl-CoA, a critical molecule in energy production. PDH serves as a bridge between glycolysis, which occurs in the cytoplasm, and the citric acid cycle (Krebs cycle), which takes place in the mitochondria.
Pyruvate Dehydrogenase Structure and Function
The PDH complex consists of multiple subunits working together to catalyze the conversion of pyruvate to acetyl-CoA. Each subunit plays a specific role in the overall function of PDH:
The first subunit, pyruvate dehydrogenase (E1), decarboxylates pyruvate and transfers the resulting acetyl group to the second subunit, dihydrolipoamide acetyltransferase (E2). E2 contains a lipoic acid cofactor that forms a transient thioester bond with the acetyl group. This acetyl group is then transferred to coenzyme A (CoA) by E2, resulting in the formation of acetyl-CoA.
The active sites of the PDH complex are crucial for its enzymatic activity. Within the E1 subunit, thiamine pyrophosphate (TPP) acts as a coenzyme, facilitating the decarboxylation of pyruvate. TPP serves as an electron sink, enabling the transfer of the acetyl group to the lipoyllysine arm of the E2 subunit.
The lipoyllysine arm, attached to E2, plays a pivotal role in transferring the acetyl group from E1 to CoA. This flexible arm shuttles the acetyl group between the active sites of E1 and E2, facilitating the transfer of the acetyl group to CoA.
The third subunit, dihydrolipoamide dehydrogenase (E3), catalyzes the reoxidation of the reduced form of the lipoyllysine arm, allowing for subsequent rounds of catalysis.
PDH operates within the mitochondria, where it acts as a link between glycolysis and the citric acid cycle. The conversion of pyruvate to acetyl-CoA by PDH is a critical step in energy production, as acetyl-CoA enters the citric acid cycle for further oxidation to generate ATP.
The Citric Acid Cycle
PDH Regulation
The activity of pyruvate dehydrogenase is tightly regulated to ensure precise control of cellular metabolism. This regulation occurs through a combination of reversible phosphorylation and allosteric modulation.
Phosphorylation is a key regulatory mechanism that modulates PDH activity. PDH kinase (PDK) phosphorylates specific serine residues on the E1 subunit of PDH, leading to the inhibition of PDH function. This phosphorylation event decreases the enzymatic activity of PDH, preventing the conversion of pyruvate to acetyl-CoA.
The phosphorylation of PDH by PDK is influenced by various factors. One critical factor is the intracellular ratio of ATP to ADP and NADH to NAD+. High levels of ATP and NADH, indicating ample energy availability, stimulate PDK activity, leading to increased phosphorylation and subsequent PDH inhibition. Conversely, increased ADP and pyruvate levels, signaling energy demand, activate PDH phosphatase (PDP) and dephosphorylate PDH, restoring its activity.
PDH and Cancer
The dysregulation of pyruvate dehydrogenase has been observed in cancer cells and is associated with the metabolic reprogramming known as the Warburg effect. The Warburg effect describes the preference of cancer cells to rely on glycolysis for energy production, even in the presence of oxygen. In many cancer cells, PDH activity is reduced, leading to a decrease in the conversion of pyruvate to acetyl-CoA. This altered regulation of PDH promotes the reliance of cancer cells on glycolysis for ATP generation, allowing for rapid energy production to support tumor growth and proliferation.
The dysregulation of PDH in cancer cells can be attributed to various factors. Increased expression and activity of pyruvate dehydrogenase kinase (PDK), an enzyme that phosphorylates and inhibits PDH, contribute to reduced PDH activity. Moreover, oncogenic signaling pathways and alterations in metabolic enzymes can also impact PDH function in cancer. The decreased activity of PDH in cancer cells leads to the accumulation of glycolytic intermediates, which can be diverted into biosynthetic pathways to support the high metabolic demands of proliferating tumor cells. This metabolic rewiring provides a growth advantage to cancer cells and plays a crucial role in tumor progression.
Targeting PDH and its regulatory enzymes has emerged as a potential therapeutic approach in cancer treatment. By restoring PDH activity or inhibiting PDK, it may be possible to shift the metabolic phenotype of cancer cells from glycolysis to oxidative phosphorylation, potentially sensitizing tumor cells to existing therapies or reducing their growth rate.
PDH Related Kits
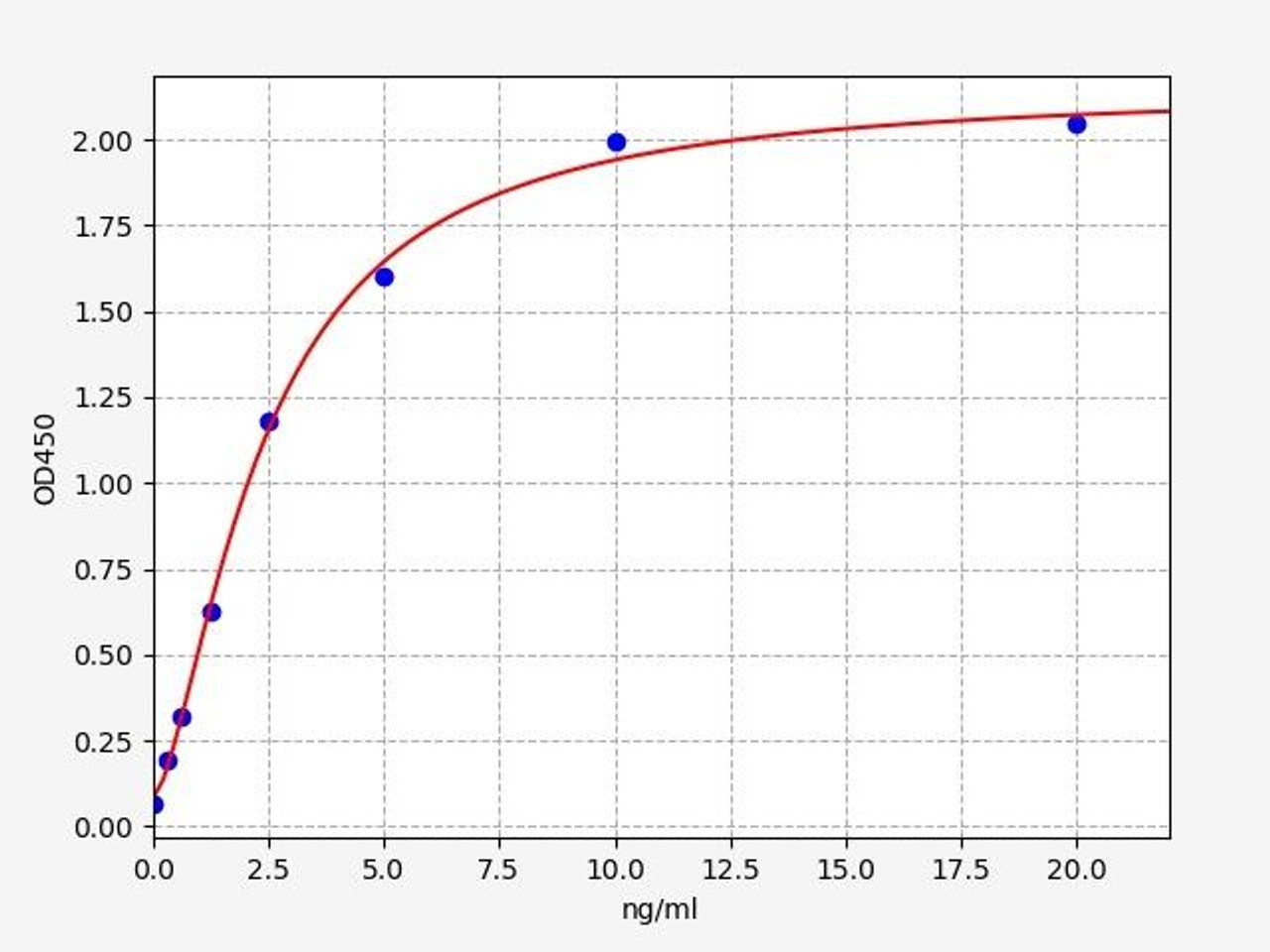
Human Pyruvate Dehydrogenase Phosphatase / PDP ELISA Kit | |
---|---|
ELISA TYPE | Sandwich |
SENSITIVITY | 0.188ng/ml |
RANGE | 0.313-20ng/ml |
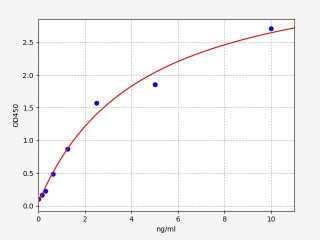
Human Pyruvate Dehydrogenase Alpha / PDHA ELISA Kit | |
---|---|
ELISA TYPE | Sandwich |
SENSITIVITY | 0.094ng/ml |
RANGE | 0.156-10ng/ml |
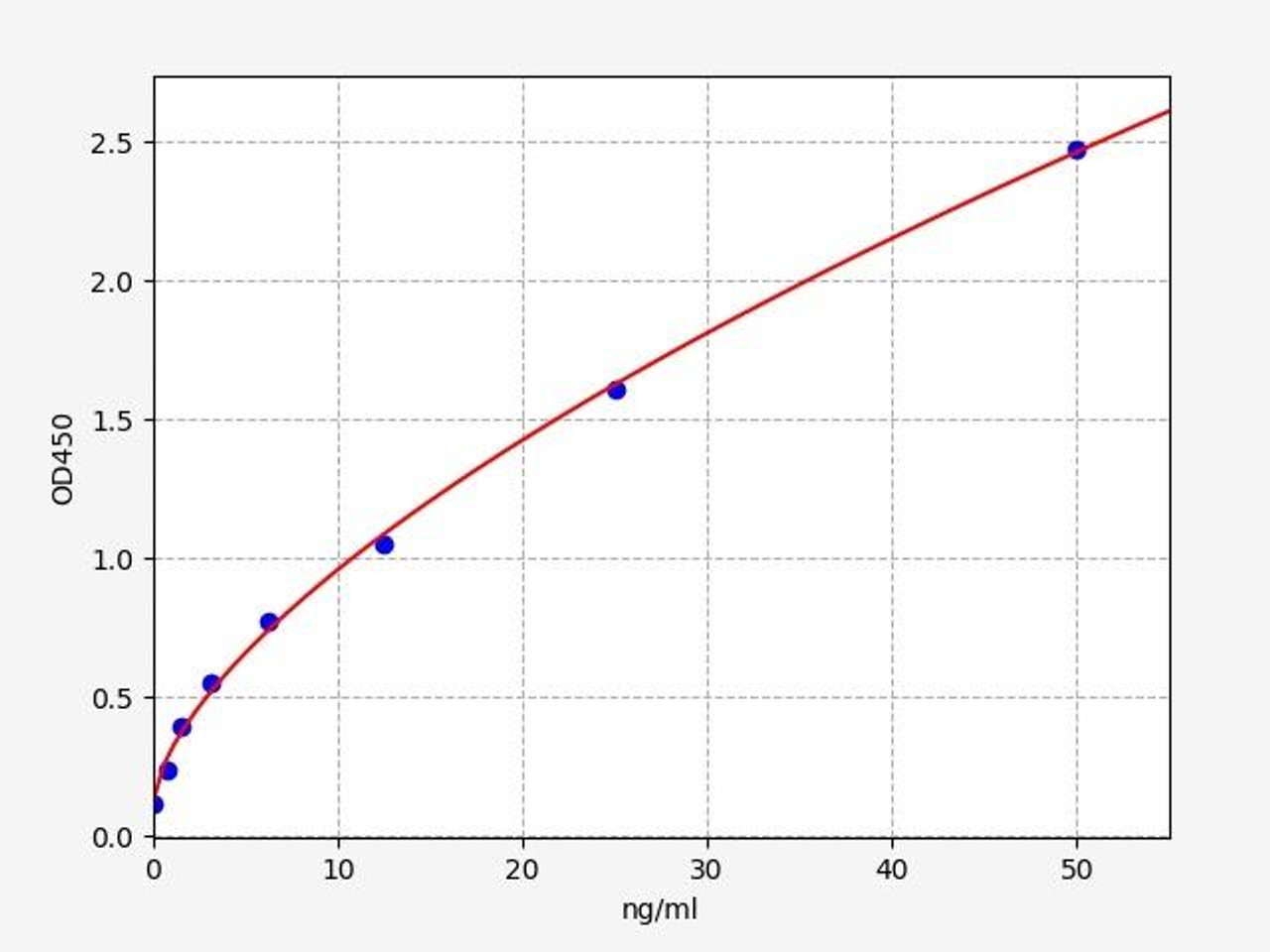
Human PKLR / Pyruvate Kinase, Liver And RBC ELISA Kit/ | |
---|---|
ELISA TYPE | Sandwich |
SENSITIVITY | 0.469ng/ml |
RANGE | 0.781-50ng/ml |
PDH and Pyruvate Dehydrogenase Complex
Dysregulation of pyruvate dehydrogenase activity has been implicated in various metabolic disorders. Pyruvate dehydrogenase complex (PDC) deficiency, a rare genetic disorder, leads to impaired PDH activity, resulting in energy production disruptions and a range of symptoms. It is characterized by the impaired activity of the PDC, leading to the accumulation of pyruvate and a decrease in the production of acetyl-CoA. PDC deficiency can manifest in various ways, including neurological symptoms, developmental delays, lactic acidosis, and metabolic abnormalities. The severity of the condition can vary, ranging from mild to severe forms. PDC deficiency is typically diagnosed through clinical evaluations, biochemical testing, and genetic analysis.
Written by Lauryn McLoughlin
Lauryn McLoughlin completed her undergraduate degree in Neuroscience before completing her masters in Biotechnology at University College Dublin.
Recent Posts
-
Enavatuzumab: Revolutionizing Cancer Research Through Novel Therapeutics
Quick Facts About EnavatuzumabWhat is Enavatuzumab?Enavatuzumab is a monoclonal antibo …17th Dec 2025 -
Alemtuzumab: Mechanism, Applications, and Biosimilar Advancements
Quick Facts About AlemtuzumabWhat is Alemtuzumab?Alemtuzumab is a monoclonal antibody …17th Dec 2025 -
Tinurilimab: Advancing Immunotherapy Through CD47 Blockade
Quick Facts About TinurilimabWhat is Tinurilimab?Tinurilimab is a monoclonal antibody …11th Feb 2025