The Essential Guide to DNA: Components, Purpose and Purification Methods
Exploring DNA's essence, this guide illuminates its structure, function, and purification techniques, essential in fields like medicine and biotechnology.
Key Takeaways:
- DNA's Structure: Composed of nucleotides adenine, thymine, cytosine, guanine, forming a double helix.
- Charge Properties: DNA exhibits a negative charge due to phosphate groups.
- Central Dogma: DNA's role in protein synthesis and inheritance.
- Extraction Methods: Techniques for isolating DNA.
- Purification Techniques: Ensuring DNA's integrity for analysis.
- Genomic DNA: The complete genetic information in cells.
- DNA Assays: Tools for DNA analysis and research applications.
Table of Contents
Jump to a section:
DNA, short for deoxyribonucleic acid, is a fundamental molecule that holds the key to life as we know it. It serves as the blueprint for the genetic information in all living organisms, from the tiniest microorganisms to complex multicellular organisms like humans. This blog focuses on the components, the purpose, extraction and purification methods for DNA. Discover its role in various fields such as medicine and biotechnology.
Understanding DNA: Components and Charge
DNA, short for deoxyribonucleic acid, serves as the fundamental blueprint of life, carrying genetic information in nearly all living organisms. To comprehend the intricacies of DNA, it is crucial to delve into its key components and the intriguing charge properties it possesses. DNA consists of four fundamental building blocks called nucleotides: adenine (A), thymine (T), cytosine (C), and guanine (G). These nucleotides come together to form the famous DNA double helix structure, where A pairs with T and C pairs with G. Remarkably, DNA exhibits an overall negative charge. This negative charge arises from the phosphate groups present in the DNA backbone, which contain negatively charged atoms. The negatively charged phosphate groups repel each other, contributing to the structural stability of DNA.
Moreover, the negative charge of DNA is of significant importance in various biological processes. It plays a crucial role in DNA replication, transcription, and repair. The negative charge of DNA allows it to interact with positively charged proteins, such as histones, which help in the compaction and organization of DNA into the intricate structure known as chromatin. Additionally, the charge properties of DNA influence its migration during gel electrophoresis, a widely used technique in DNA analysis.
Understanding the components and charge of DNA unveils the fascinating nature of this molecule and lays the foundation for exploring its profound role in genetics and inheritance. By deciphering the language embedded within DNA, scientists have unlocked invaluable insights into the inheritance of traits, the development of organisms, and the mechanisms underlying genetic disorders. This understanding has paved the way for advancements in fields such as personalized medicine, genetic engineering, and forensic analysis, shaping our understanding of life itself.
DNA Structure
DNA Functions: Exploring Its Purpose
DNA is a versatile molecule with a multitude of uses and roles. Its purpose lies in serving as the repository of genetic information, carrying the instructions necessary for the development, functioning, and inheritance of all known organisms. At its core, DNA acts as a blueprint for the synthesis of proteins, the workhorses of cellular activities. Through the process of transcription and translation, the genetic code stored in DNA is transcribed into RNA and ultimately translated into proteins, which govern the structure and function of cells and tissues. Moreover, DNA plays a pivotal role in inheritance, passing on genetic traits from one generation to the next.
The functions of DNA extend beyond protein synthesis and inheritance. DNA also serves as a valuable tool in scientific research and technological applications. It can be harnessed for various purposes, such as genetic engineering, where specific genes can be manipulated or inserted into an organism's DNA to confer new traits or produce beneficial compounds. DNA analysis techniques, such as DNA sequencing and fingerprinting, have revolutionized fields like forensic science and molecular diagnostics, enabling the identification of individuals, detection of genetic diseases, and the exploration of genetic diversity.
The Central Dogma Theory
The Central Dogma of molecular biology is a foundational concept that elucidates the flow of genetic information within living organisms. It outlines the fundamental processes through which genetic instructions are transferred from DNA to RNA and eventually translated into proteins. The nucleotides join together in a specific sequence to form the famous double helix structure of DNA. The sequence of nucleotides within DNA encodes the instructions required for the synthesis of proteins, the key players in various cellular functions.
Central Dogma Theory
Replication
DNA replication is a remarkable process that ensures the faithful transmission of genetic information from one generation to the next. In both prokaryotic and eukaryotic cells, DNA replication follows a highly orchestrated series of steps, each essential for the accurate duplication of the genetic material. The replication process begins with the unwinding of the DNA double helix, facilitated by specialized enzymes known as helicases. Once the strands are separated, DNA polymerases come into action, synthesizing new DNA strands complementary to the original template strands. In prokaryotes, such as bacteria, DNA replication proceeds bidirectionally from a single origin of replication, resulting in two replication forks moving in opposite directions. In contrast, eukaryotes, including plants, animals, and humans, undergo replication at multiple origins, allowing for faster replication across their larger and more complex genomes. The significance of DNA replication is profound, as it serves as the foundation for cell division, growth, and genetic stability.
Transcription
DNA transcription is a fundamental process that plays a pivotal role in the expression of genetic information in both prokaryotic and eukaryotic organisms. It involves the synthesis of RNA molecules from DNA templates and serves as a critical step in gene expression. The process of DNA transcription consists of several distinct steps, each contributing to the accurate transfer of genetic instructions. In prokaryotes, which include bacteria and archaea, transcription occurs within the cytoplasm. The initial step, known as initiation, involves the binding of RNA polymerase to the promoter region on the DNA template. Subsequently, the polymerase unwinds the DNA double helix and begins the elongation phase, synthesizing an RNA molecule complementary to the DNA template strand. Finally, termination signals halt the transcription process, resulting in the release of the newly synthesized RNA molecule.
Similarly, in eukaryotes, which encompass plants, animals, fungi, and protists, DNA transcription takes place within the nucleus. However, it is a more complex process compared to prokaryotic transcription. Eukaryotic transcription involves the participation of three different RNA polymerases: RNA polymerase I, II, and III, each responsible for transcribing specific types of RNA molecules. Additionally, the process requires the involvement of transcription factors and regulatory elements to ensure precise control and regulation of gene expression. This intricate orchestration allows eukaryotic cells to finely modulate gene activity in response to various environmental cues and developmental stages.
Understanding the steps and significance of DNA transcription in both prokaryotes and eukaryotes is crucial for unraveling the intricate mechanisms underlying gene regulation and protein synthesis.
Translation
DNA translation is a fundamental process in both prokaryotes and eukaryotes that plays a vital role in protein synthesis, the central mechanism by which genetic information encoded in DNA is transformed into functional proteins. This intricate process involves a series of well-coordinated steps that occur within the cellular machinery. The first step, known as initiation, entails the binding of ribosomes to the messenger RNA (mRNA) molecule. In prokaryotes, initiation requires the recognition of a specific sequence called the Shine-Dalgarno sequence within the mRNA, while in eukaryotes, initiation involves the recognition of the 5' cap structure on the mRNA. Following initiation, the ribosome proceeds to the elongation phase, where transfer RNA (tRNA) molecules carrying amino acids are sequentially brought to the ribosome. The ribosome reads the codons on the mRNA, and the tRNA molecules with complementary anticodons bind to the codons, allowing for the assembly of the growing polypeptide chain. This stepwise addition of amino acids continues until a stop codon is reached, signaling the termination of translation. The significance of DNA translation is immense, as it is responsible for the synthesis of proteins that govern various biological processes, including enzymatic activities, structural components, and regulatory functions within cells.
Table 1. Differences between Eukaryotes vs Prokaryotes DNA synthesis
Organisms | Replication | Transcription | Translation |
Eukaryotes | Requires multiple replication forks to replicate the entire genome | Transcription occurs in the nucleus. RNAs are released and processed in the nucleus | Asynchronous process |
Prokaryotes | Requires only a single origin to replicate the entire genome | Transcription occurs in the cytoplasm RNAs are released and processed in the cytoplasm. | Synchronous process |
DNA Extraction: Methods and Buffers
DNA extraction is a fundamental process in molecular biology that allows scientists to isolate DNA from various biological samples for further analysis. It is a crucial step in many research areas, including genetics, genomics, forensics, and medical diagnostics. Effective DNA extraction involves the utilization of appropriate methods and buffers to ensure the isolation of high-quality DNA.
One commonly employed method for DNA extraction is column-based purification. In this technique, a DNA extraction column is used as a solid support matrix. The column contains specialized materials that allow for efficient binding of DNA molecules while removing impurities. During the extraction process, the biological sample, which may be a tissue, blood, or cells, is lysed to release the DNA. The lysate is then loaded onto the column, and the DNA selectively binds to the column matrix while unwanted cellular debris and contaminants pass through. By using this method, researchers can obtain DNA that is free from impurities, ensuring accurate downstream analysis.
The choice of buffers is another crucial aspect of DNA extraction. Buffers are solutions that help create and maintain the optimal environment for the extraction process. One essential component is the DNA extraction buffer. This specialized solution is designed to lyse or break open the cells, releasing the DNA from within. It typically contains a carefully formulated combination of salts, detergents, and other reagents that aid in cellular disruption and stabilize the released DNA. The DNA extraction buffer's composition is carefully optimized to promote efficient DNA recovery while minimizing degradation and contamination.
In addition to the DNA extraction buffer, a DNA extraction solution is often used in the purification steps following DNA release. This solution helps facilitate the separation and purification of the DNA from other cellular components. It aids in the removal of proteins, lipids, and other impurities that might interfere with downstream applications. The DNA extraction solution is formulated to maintain specific pH and ionic strength conditions, ensuring the stability and integrity of the DNA during the purification process.
Throughout the DNA extraction procedure, the DNA buffer plays a critical role in maintaining the optimal conditions for DNA preservation. It helps regulate the pH and ionic strength of the extraction system, creating an environment where DNA remains stable and intact. By carefully controlling these factors, scientists can prevent the degradation of DNA and preserve its structural and functional integrity.
Related Products
DNA Related Kits

Genie Fusion Ultra High-Fidelity DNA Polymerase | |
---|---|
SKU | MORV0001 |
Storage | Store at -20℃ |
Applications | Apmlification of gDNA, crude samples |
Genie Fusion Ultra High-Fidelity DNA Polymerase is a next generation enzyme for robust PCR with ultra high-fidelity.
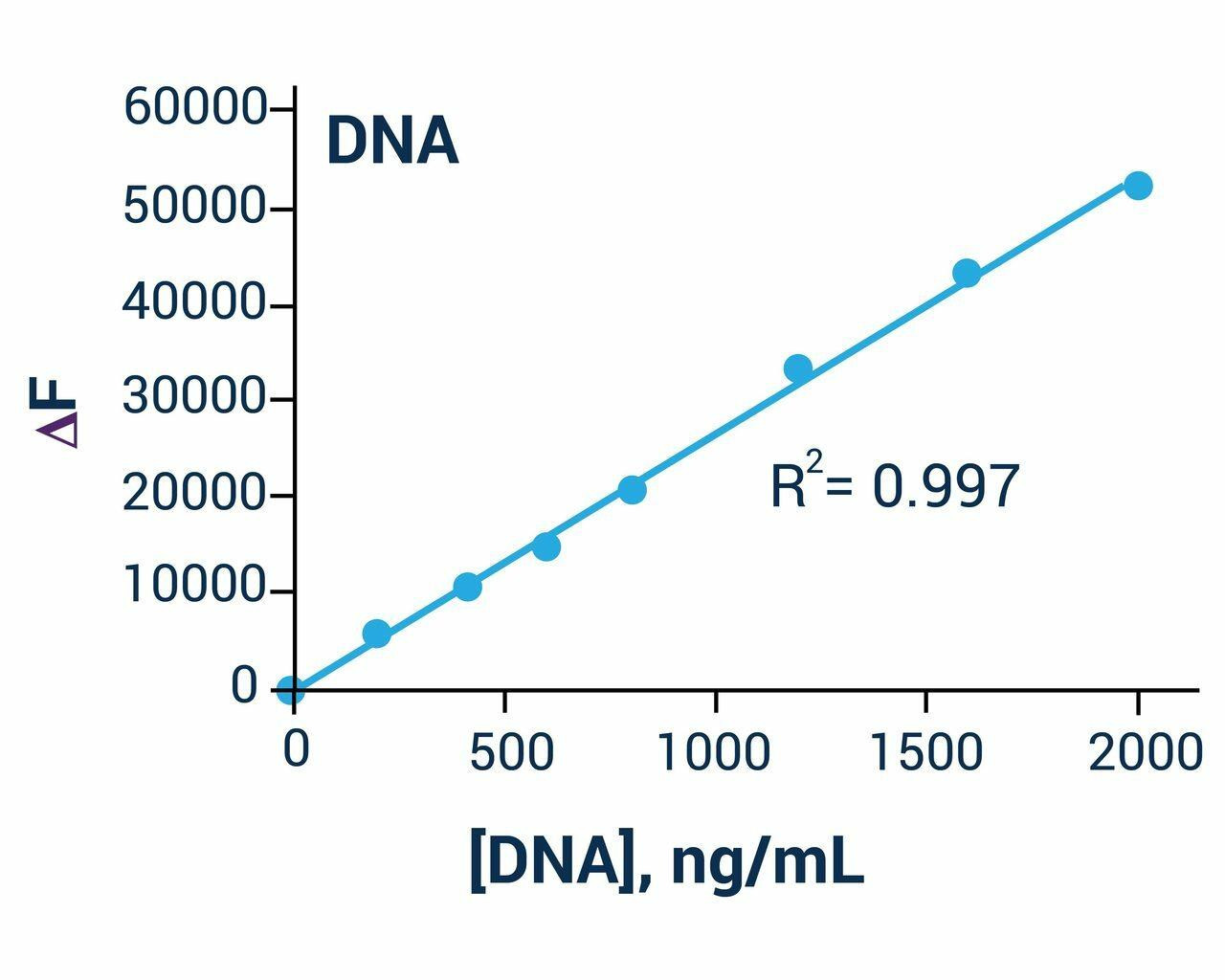
DNA Assay Kit (Fluorometric) (BA0171) | |
---|---|
SKU | BA0171 |
Storage | DNA standard at -20°C, Reagent at 2-8°C. |
Applications | Qantitative determination of DNA. |
Assay Genie's Fluorometric DNA assay kit is designed to accurately measure nanogram quantities of plasmid DNA, cDNA, DNA following polymerase chain reaction and DNA eluted from gels
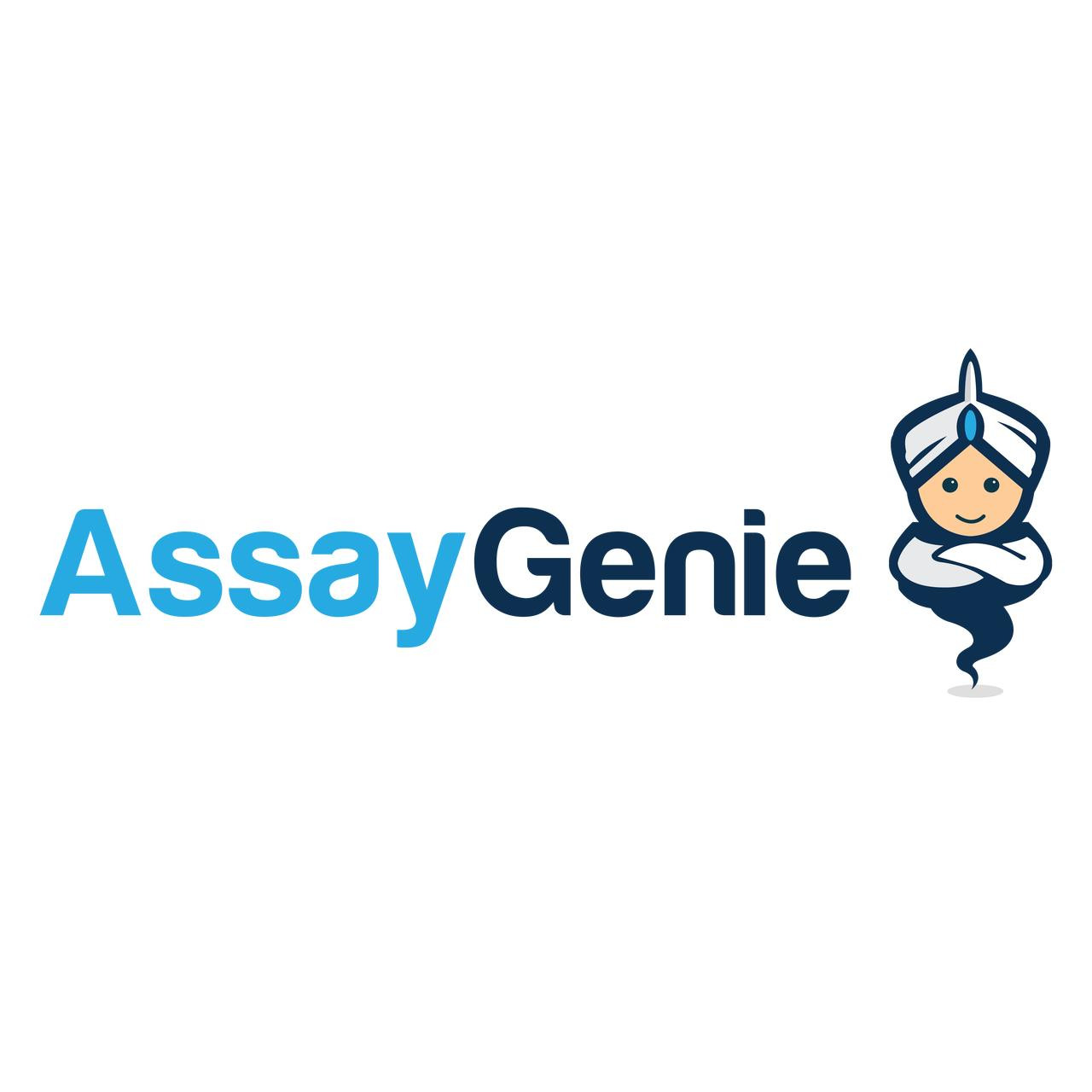
GenieClone DNA Assembly Cloning Kit | |
---|---|
SKU | MORV0004 |
Storage | All components at -20℃ |
Applications | Fast Cloning, Seamless Assembly |
GenieClone DNA Assembly Cloning Kit allows for the highly efficient cloning and assembly of 1-5 DNA fragments in as little as 15 minutes into any vector at any site.
DNA Purification Techniques
DNA purification techniques are essential processes used in scientific research to isolate DNA from complex biological samples with the aim of obtaining high-quality DNA samples for further analysis. One of the key components in DNA purification is the utilization of a centrifuge, which plays a vital role in DNA extraction.
A centrifuge is a laboratory instrument that employs the principles of centrifugal force to separate different components within a sample based on their density. In the context of DNA extraction, the centrifuge is used to separate DNA molecules from other cellular components, such as proteins, lipids, and cellular debris. The centrifuge achieves this separation by subjecting the DNA-containing sample to high-speed rotation, which generates a centrifugal force that drives the denser DNA molecules to migrate towards the bottom of the centrifuge tube.
During the centrifugation process, the cellular components separate into distinct layers within the tube. The DNA, being relatively denser, forms a pellet at the bottom of the tube, while the lighter components remain in the supernatant. Once the separation is complete, the supernatant is carefully removed, leaving behind the DNA pellet.
Following DNA extraction, the isolated DNA undergoes chemical analysis to assess its quality, concentration, and purity. Chemical analysis techniques, such as spectrophotometry, fluorometry, or agarose gel electrophoresis, are employed to determine various characteristics of the DNA sample.
Spectrophotometry is a widely used technique that measures the absorbance of DNA at specific wavelengths of light. This measurement provides information about the DNA concentration and purity by assessing the presence of contaminants, such as proteins or other impurities, which can affect the accuracy of downstream applications.
Fluorometry, on the other hand, utilizes fluorescent dyes that bind specifically to DNA molecules, allowing for the quantification of DNA concentration. This method is highly sensitive and provides precise measurements of DNA concentration, often requiring smaller sample volumes.
Agarose gel electrophoresis is a technique used to visualize and separate DNA fragments based on their size. By applying an electric field to a gel matrix containing the DNA sample, the DNA molecules migrate through the gel at different rates, resulting in distinct bands that can be visualized under UV light. This technique helps determine the size range of DNA fragments and assess their integrity.
Genomic DNA : Function and Analysis
Genomic DNA, also known as gDNA, plays a fundamental role in the inheritance and expression of genetic information within an organism. It is the hereditary material present in the nucleus of each cell and holds the complete set of genetic instructions required for the development, growth, and functioning of an organism. The function of genomic DNA lies in its ability to encode and store vital genetic information, including the instructions for protein synthesis, regulation of cellular processes, and inheritance of traits across generations. Analyzing genomic DNA provides valuable insights into the structure, organization, and variations within an individual's genetic makeup, enabling scientists to unravel the complexities of life at a molecular level and explore the underlying mechanisms of genetic diseases, evolutionary relationships, and population genetics.
In conclusion, understanding the essential components of DNA, the intricate process of DNA replication, and the various purification methods is crucial for unraveling the mysteries of life and advancing scientific knowledge. The intricate structure of DNA, comprised of nucleotides and double helix configuration, forms the foundation for genetic information storage and transmission. DNA replication ensures accurate inheritance and plays a pivotal role in cellular division. Lastly, the purification methods discussed provide researchers with the tools to isolate and extract high-quality DNA samples, enabling further analysis and unlocking a deeper understanding of genetic processes. By understanding the basics of DNA, we gain invaluable insights into the complexities of life's blueprint, paving the way for advancements in fields such as medicine, agriculture, and biotechnology.
Written by Pragna Krishnapur
Pragna Krishnapur completed her Bachelor degree in Biotechnology Engineering at Visvesvaraya Technological University before completing her Masters in Biotechnology at University College Dublin.
Recent Posts
-
MHC Class I vs MHC Class II: Key Differences and Functions
Major Histocompatibility Complex (MHC) molecules are essential for immune recognition …22nd Nov 2024 -
Amino Acids: Functions, Roles, and Structures
Amino acids are the fundamental units of proteins, playing critical roles in virtually …22nd Nov 2024 -
Immunoglobulins: Structure, Function, and Clinical Importance
Immunoglobulins (Igs), also known as antibodies, are glycoproteins produced by B cells …20th Nov 2024